Chemistry 114
Alkanes and Cycloalkanes
Structure and Properties
General formula: CnH2n+2
All carbon-carbon and carbon-hydrogen single bonds (saturated)
Tetrahedral arrangement around each carbon
sp3 hybridized
Free rotation around single bonds
Boiling points of alkanes
Increase with increasing carbon number (increased molecular weight) [See
Figures 15.2 and 15.6]
At room temperature (RT) and 1 atm pressure
- C1 to C4 are gases
- C5 to C17 are liquids
- C18 and higher are solids
Increased boiling points (and melting points) are due to stronger intermolecular
forces.
In alkanes the only significant intermolecule force is the VanderWaals interaction
(aka London dispersion forces or induced dipoles). This attractive force
results from charge-charge interactions between molecules due to transient
uneven distributions of electrons. Extra electron density in one part of
a molecule (resulting in slight negative charge) repels the electrons density
in a neighboring molecule (resulting in a slight positve charge). The positive
and negative charges attract. The more carbons there are in a hydrocarbon
(i.e. the higher the molecular weight) results in more electrons that produce
a more significant intermolecular interaction.
Branched alkanes have a lower boiling point than their corresponding unbranched
isomer. Branching produces less efficient packing and thus weakens the intermolecular
interactions.
Solubilities of alkanes
Very low solubility in aqueous solvents (immiscible). Alkanes (and other
organic solvents) tend to separate into a separate layer when mixed with
water.
Since C-H groups are not hydrogen bond donors, alkanes disrupt the hydrogen
bonding network of water. Also, when a non-polar molecule is incorporated
into an aqueous solution, the waters form a rigid molecular cage around
the non-polar molecule. This is comparable to freezing and is an unfavorably
process due to the reduced entropy (disorder).
Like dissolves like. Alkanes are very soluble in other organic solvents.
Nomenclature of alkanes
Straight chained hydrocarbon (and parent carbon chains): prefix for the
number of carbons + -ane
For a subsituent group based on an alkane (an alkyl group): prefix for the
number of carbons + -yl
Know all of the prefixes listed in this table.
Number of Carbons Prefix alkane alkyl group
1 meth- methane methyl
2 eth- ethane ethyl
3 prop- propane propyl
4 but- butane butyl
5 pent- pentane pentyl
6 hex- hexane hexyl
7 hept- heptane heptyl
8 oct- octane octyl
9 non- nonane nonyl
10 dec- decane decyl
Special alkyl grops that you must know (see Tables 15-2
and 15-3):
- isopropyl
- sec-butyl
- isobutyl
- tert-butyl
General Rules of Nomenclature for Alkanes (IUPAC)
1. The longest continuous carbon is the parent compound. The base name for
the molecule is the prefix for the number of carbons of the parent compound
+ the suffix -ane. Number the carbons in the parent chain so that substituents
have the smallest possible numbers.
2. Find each alkyl substituent and assign it a number according to which
carbon it is attached.
3. List the substituents (with their carbon number) in alphabetical order
followed by the name of the parent alkane. If there are more than one of
a given substituent use the prefixes di-, tri-, tetra-. Prefixes
are not used to determine alphabetical order.
4. Use commas between numbers and dashes between numbers and words. Do not
leave spaces in the name.
Examples:
- 2,2-dimethylpropane
- 4-ethyl-2,3-dimethyloctane
Nomenclature for Organohalogens
1. Organohalogens are named just like their parent compound alkane with
the halogen treated exactly as alkyl substituents.
2. -F, -Cl, -Br and -I substituents are labeled fluoro-, chloro-, bromo-
and iodo- , respectively.
Nomenclature for Cycloalkanes
1. Cycloalkanes are alkanes in which a bond is formed between the two terminal
carbons in the chain to for a cyclical or ring structure. The prefix cyclo-
is written before the name designating the carbon number in the ring (e.g.
cyclohexane).
2. Substituents are named similarly to straight-chained alkanes. The carbons
in the ring are numbered so that the substituents have the smallest numbers.
3. Because the ring structure prevents free rotation around the C-C bonds,
there is a spatial difference between above the plane of the ring and below
the plane of the ring. If two substituents are attached to two different
carbons on opposite sides of the plane of the ring, they are said to be
trans; if they are on the same side of the plane of the ring, they
are said to be cis. If the plane (or approximate plane) of the ring
is drawn in the plane of the drawing surface, then a substituent coming
up out of the plane is indicated by a thick wedge-shaped bond; a substitutent
going into the plane is indicated by a dotted line. The cis or trans
label is written at the beginning of the compounds name. The figure below
illustrates several of these concepts.
Molecule A is trans-1,3-dimethylcyclohexane.
Molecule B is cis-1,3-demethylcyclohexane.
The carbon chain ring of most cycloalkanes is not planar (cyclopropane must
be and cyclobutane is very close). Because of free rotation around the single
bonds, the molecules adopt a shape so that the bond angles are as close
as possible to 109.5. See the discussion of conformation below.
Conformation of alkanes and cycloalkanes
Because of free rotation around C-C single bonds atoms or groups attached
to the bond carbons can be in various spatial relationships to one another.
These different spatial arrangements are known as different conformations.
This is a very important topic in chemistry and biochemistry. In proteins,
for example, the difference between a folded, biologically functional protein
and an unfolded, denatured, inactive protein is simply a change in conformation,
i.e. rotations around single bonds (although very many of them in a molecule
as large as a protein.)
Ethane
The easiest way to show differences in conformation is by looking down the
C-C bond being rotated and determine the relative positions of attached
atoms or groups. This is visualized with the Newman projection illustrated
in the figure below for ethane.
In each figure the structure on the left represents a 3D model of ethane
whereas the structure on the right is the Newman projection. Figure A
is known as the eclipsed conformation because the viewed down the C-C bond
the hydrogens are aligned. Figure B is known as the staggered
conformation. The staggered conformation is the lower energy (more favorable
conformation) because the H atoms and the C-H bonds one one carbon are less
crowded with the H atoms and the C-H bonds on the other carbon. Figure 15.13
in the textbook shows the relative energies of the conformations as the
molecule is rotated around the C-C bond.
Butane
Rotation around the central C-C bond of butane is a bit more complicated.
It can be treated like ethane but with a methyl group (-CH3)
replacing an H on both the front and the back carbon. The main Newman projections
for butane are shown in the figure below.
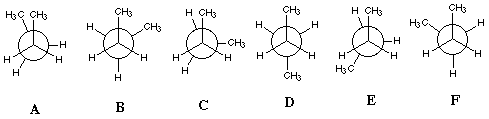
Notice that the front methyl group is unchanged in all of these structures.
Conformations A, C and E are
eclipsed, whereas conformations B, D and
F are staggered. A is the highest energy
(least favorable) conformation because of crowding between the two methyl
groups when they are eclipsed. It is known as the syn conformation.
D is the lowest energy (most favorable) conformation because
the two methyl groups are farthest apart. This is know as the anti conformation.
B and F are low energy conformations but
not as low energy as the anti. They are known as gauche+ and
gauche-. C and E are high energy
conformations but not as high as the syn conformation.
Cyclohexane
Rotation ar